Advanced Sheet Metal Fabrication Technology
Advanced Sheet Metal Fabrication Technology
By: CapableMaching
Preface:
Sheet metal fabrication is essential due to its versatility, cost-effective manufacturing for high-volume production, lightweight yet strong properties, ability to provide precise customization, suitability for quick prototyping, provision of high-quality surface finishes, durability, efficient material usage, high-speed production, ease of assembly, capability to handle complex geometries, and widespread applicability across various industries.
Sheet metal fabrication, compared to the more general mechanical processing, in the eyes of the common people seems to be not so much advanced technology sheet metal fabrication has many advanced forming technologies, especially in the aircraft aviation manufacturing industry. Aircraft need sheet metal working technology for a variety of reasons. This technology plays a key role in aircraft manufacturing, responding to complex design requirements and producing strong, lightweight structures that improve the performance and aerodynamics of the aircraft. Sheet metalworking also enables the manufacturing process to realize the diversity of aircraft shapes to meet different aerodynamic and spatial configurations. In addition, the application of this technology enables lightweight design, which is critical to improving the fuel efficiency of aircraft.
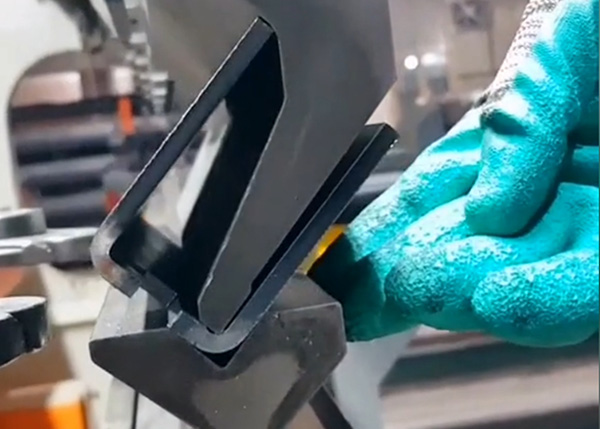
The development trend of aircraft is toward a high thrust-to-weight ratio, low fuel consumption, long service life, and low cost. Due to the extensive use of new materials, and new overall and lightweight complex structures, the forming technology of sheet metal parts has also put forward higher requirements and new challenges. According to statistics, the number of sheet metal parts in aircraft engines reaches more than 30% of the total number. The manufacturing materials of these sheet metal parts are usually high-strength, highly corrosion-resistant high-temperature alloys, titanium alloys, etc., to meet the complex working conditions of aero-engines in high-speed, high-temperature environments. They serve as the main building blocks of the aero engine and are responsible for supporting and protecting other parts of the engine.
Aero-engine sheet metal parts manufacturing enterprises are generally small batch, multi-machine production, and processing mode, the main processing methods for cold forming and hot forming two categories.
At present, the aero-engine host plant sheet metal fabrication process in addition to press bending, roll bending, sinking, deep drawing, flanging, calibration, and other common processes, also includes hot forming technology, superplastic molding technology, diffusion joining technology, high-pressure molding technology within the tube, plate liquid filling molding technology and free bending of tubes molding technology, etc., the stability of the parts in the processing process, consistency and high requirements. How to meet the growing demand for aero-engine manufacturing, it is necessary to put forward new requirements and explore new process methods.
This paper pin some of the current advanced sheet metal parts forming technology, including concepts, methods, equipment, applications, and development levels.
1. Incremental Forming technology
Incremental Forming forming is a moldless metalworking process that relies on the deformation tool to apply pressure to the local deformation to obtain the desired shape of the process, also known as incremental sheet metal forming (ISF) [1]. As one of the latest sheet metal processing technologies, it has been a hot topic of research in recent years, and the technology is currently used in several industries such as aerospace, automotive, and custom product manufacturing.
Incremental Forming technology has the advantages of low-cost molds (or no molds), fast response forming, high forming limits, the trajectory can be changed in real-time, low energy consumption, and green forming. Incremental Forming according to the presence or absence of molds and the number of moving tools divided into single-point incremental forming (Single Point Incremental Forming, SPIF), double-point incremental forming (Two Point Incremental Forming, TPIF), double-sided incremental forming (Double-sided Incremental Forming, TPIF), double-sided incremental forming, and double-sided incremental forming. sided Incremental Forming, DSIF) [2], as shown in Figure 1.
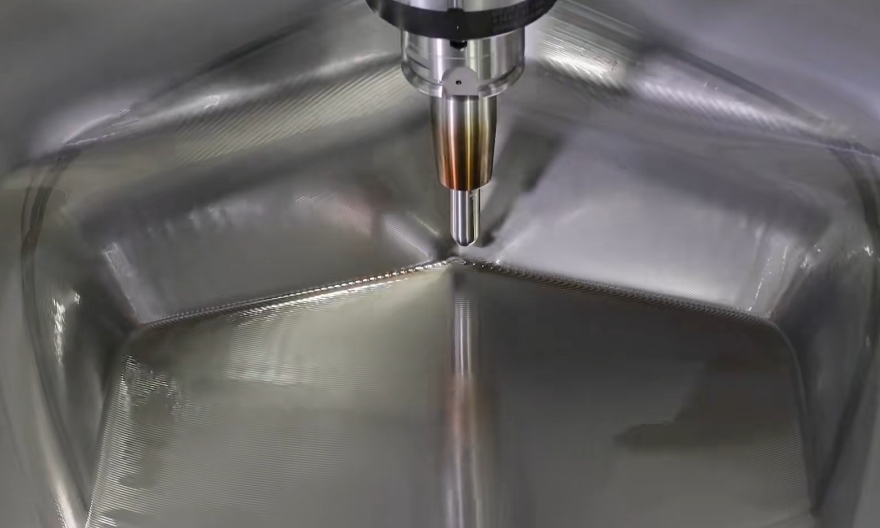
Figure 1
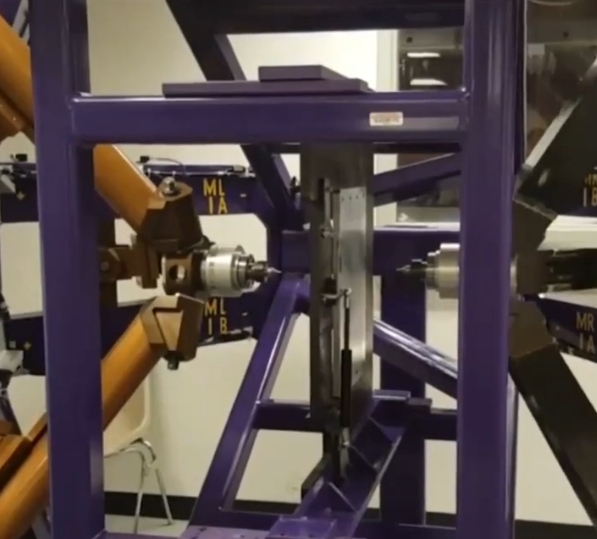
Figure 2
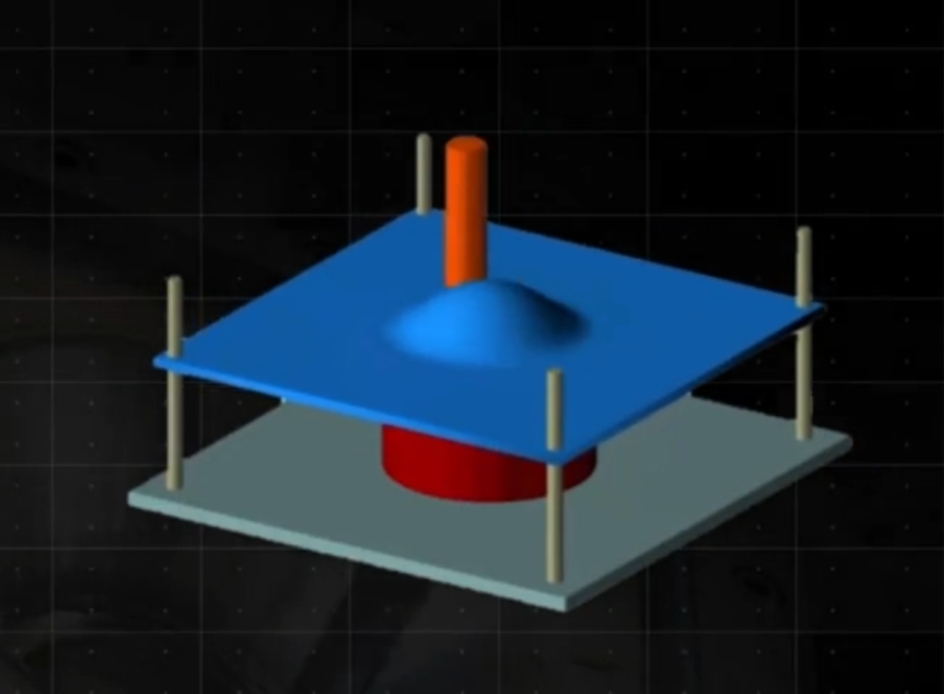
Figure 3
2. Servo vibration-assisted deep drawing technology
In the early 1950s, vibration was introduced into the field of metal plastic forming, forming an emerging cross-discipline. A large number of studies and process experiments show that vibration-assisted deep-drawing forming has volume and surface effects, which will have an impact on the internal stress of the material, and the friction between the mold and the specimen. Vibration-assisted deep-drawing technology has significant effects in reducing forming force, reducing friction, reducing flow stress, reducing spring back, improving the drawing ratio, improving the surface quality of the workpiece, and suppressing wrinkling and rupture [3-8].
Vibration-assisted deep drawing forming process is divided into ultrasonic vibration-assisted deep drawing and low-frequency vibration-assisted deep drawing two kinds. For smaller parts, often using ultrasonic vibration-assisted deep drawing. Because the power of ultrasonic vibration is relatively small, its vibration energy can not be transferred to the entire blank, and ultrasonic vibration in the large deep drawing forms easy to occur “leakage” phenomenon. In recent years, with the rapid development of servo press technology, for larger parts, often using low-frequency vibration-assisted deep drawing process [9].
Germany S.DUNKESGmbH developed servo screw press (Servo-screwPress) ES4-160/120 shown in Figure 4, using servo motors and screw drive mechanism to achieve high-precision torque and pressure control, vibration can be added to the convex die, concave die and crimp ring [10].
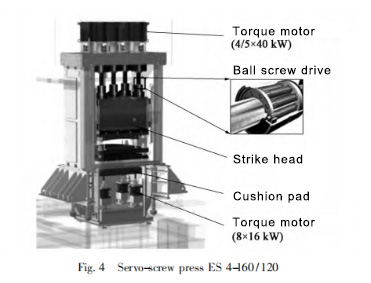
Figure 4
As shown in Fig. 5 and Fig. 6, the deep drawing depth can be increased by 1 times by motor servo vibration crimping, and the deep drawing depth can be increased from 82mm in conventional deep drawing Fig. 4 Servo-screw press ES4-160/12046mm to 82mm in vibration deep drawing [11].
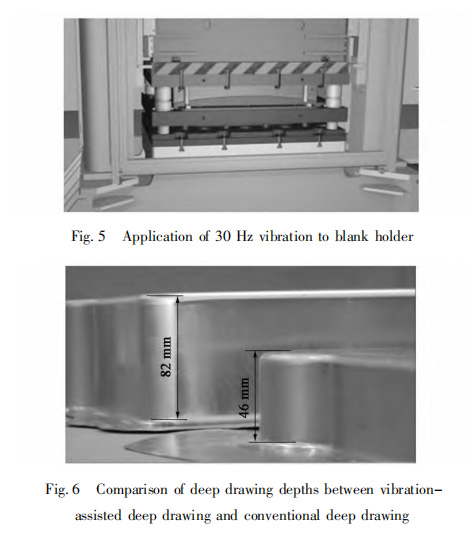
3. Rubber molding technology
Rubber forming technology includes two types rubber pad forming technology and rubber bladder forming technology. Rubber pad forming process, half-mold (convex or concave mold), and blanks placed on the table, the table into the hydraulic press. The rubber cushion (multi-layer rubber) and its capacity frame are fixed in the upper end of the hydraulic press, the table on the rubber cushion pressure. Under high pressure, the rubber pad exhibits fluid properties, and the whole surface of the part applies uniform pressure. The rubber pad-forming process is shown in Figure 7 [12]. Where the arrow is the direction of force on the blank.
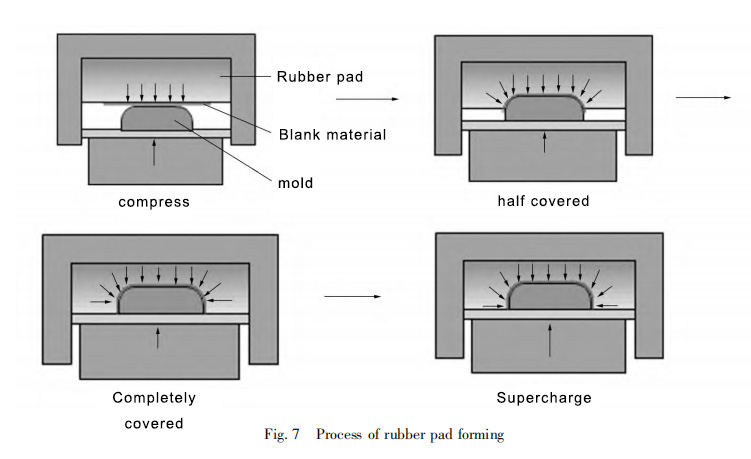
France ACB company, the United States CyrilBath company produced rubber mat forming machine tools, with the maximum tonnage of 20000t, and the maximum forming pressure of 80MPa [13].
The capacity frame and table of the rubber bursa-forming hydraulic machine form a sealed space. Forming die, blanks and rubber used as a general-purpose half-mold is placed in this space. Installed in the frame used as a general half-mold with the rubber is not an iteration of solid rubber, but a piece of thick rubber (outer tire) and an oil-filled rubber bladder (also often referred to as the inner tube) composed of two parts. To the bag into the high-pressure oil, high-pressure oil so that the rubber bladder expands, thus forcing the rubber tire to fill with all the space within the table, the blanks attached to the forming mold. After unpressurization, the table needs to be pulled out of the machine frame before the blank and mold can be replaced. The process is shown in Figure 8.
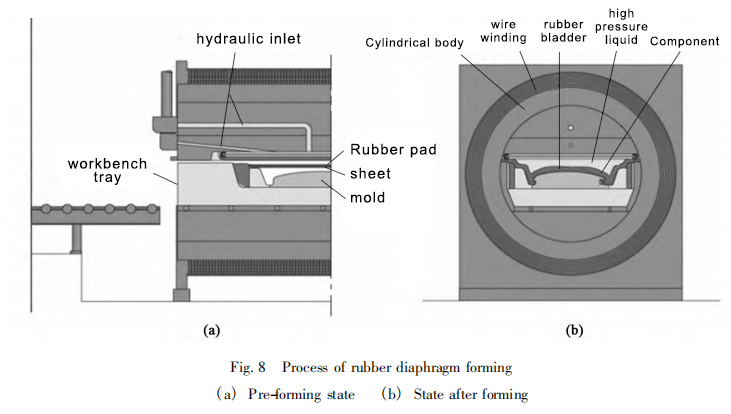
A new High-Pressure WarmForming (HPWF) technology has been developed by the Swedish company QUINTUS to form thin-walled parts of aerospace-grade titanium alloys at temperatures lower than those of hot forming, hot stamping, and superplastic molding. This combination of high pressure and high temperature not only increases forming speed and reduces cost, but also improves the forming accuracy of Ti6Al4V titanium alloys. The method introduces an induction heating system so that the blank and die are heated to approximately 270°C before entering the press and are formed at a pressure of 140 MPa. The HPWF process has the advantages of no need for protective gases, short lead time, low die cost, and low energy consumption. Figure 9 shows a typical flow of the HPWF process, in order:
- mold preheating;
- billet preheating;
- induction heating of billet and mold;
- high-pressure forming;
- cooling of the part;
- heat treatment (as required);
- thermal calibration (as required).
This process is very suitable for titanium alloy curved edges and shallow deep-drawing parts forming, forming the typical parts, and their spring back deviation after forming is shown in Figure 10, the visible parts of the forming spring back deviation are less than 0.5mm [14].
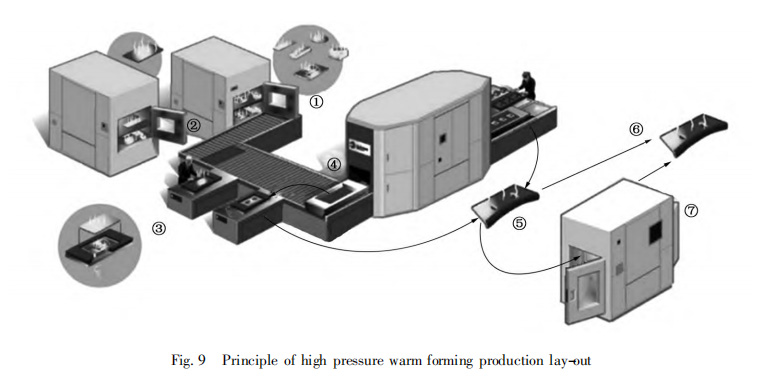
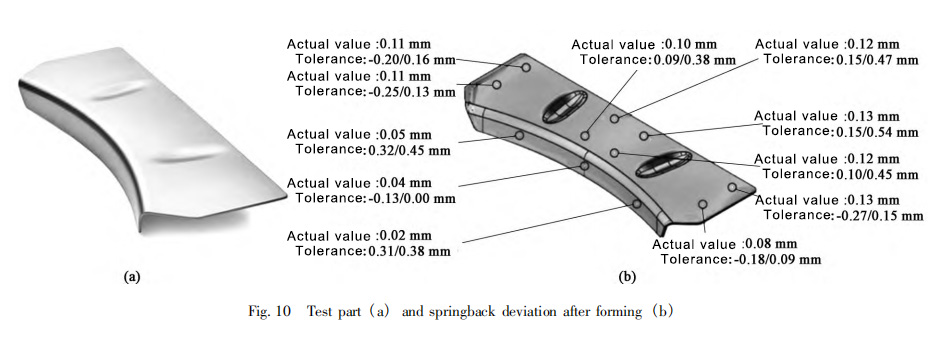
4. Hydroforming technology
According to different forming objects, hydroforming can be divided into tube hydroforming and plate hydroforming two processes.
The pipe hydroforming process, the essence of the process that the pipe in the axial feed and hydraulic expansion of the coordinated role of forming the required parts, is divided into multi-pass pipe forming, straight pipe expansion, bending pipe expansion, hydraulic punching, hydraulic expansion connection and hydraulic calibration and other 6 categories. Plate hydroforming can be divided into active Hydroforming and passive hydroforming. The active hydroforming process is shown in Figure 11 [15], passive hydroforming process is shown in Figure 12, where the arrow is the pressure on the workpiece during Hydroforming.
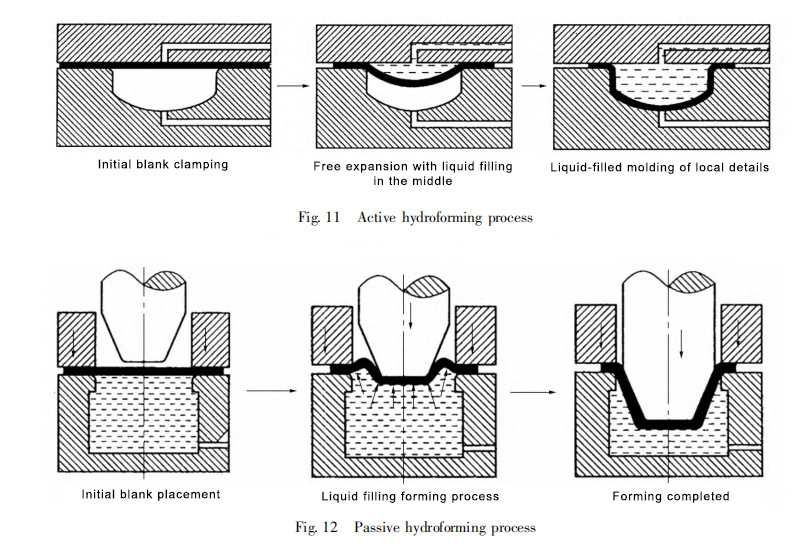
Germany’s Schuler, Sweden’s AP & T, and Japan’s Amino North America have developed a Hydroforming equipment.
General Dynamics (GeneralDynamics, GD.US) used liquid-filled molding technology to form parts on GE90-115, CFM56, GET700, and other types of engines. As shown in Fig. 13, the lip of the GE90 series engine for Boeing 777 was processed by Hydroforming, and loaded for application and mass production, with an outer diameter of about Φ3600 mm and an inner diameter of about Φ2800 mm.
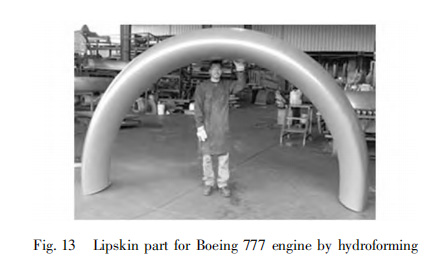
5. Axial Load BulgeForming Technology
Axial Load Bulge Forming is an innovative machining process, that uses internal hydraulic pressure to generate circumferential force to expand the metal material into the master mold, to achieve uniform wall thickness and complex geometric shapes, the principle of which is shown in Figure 14.
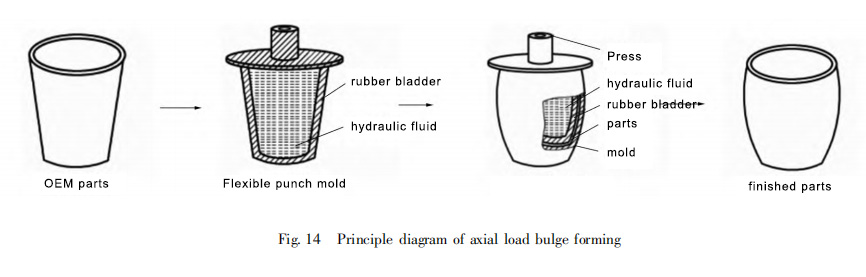
Exotic Metals Forming Company (EMFCO) designed its own 2500t expansion press with automatic loading and unloading functions for loading and unloading of large-diameter parts and heavy-duty tooling. The process is not only suitable for small parts, but EMFCO has successfully applied it to the machining of large parts with a diameter of more than Φ2032mm. In addition, the process can be used to create a wide range of different geometries through innovative designs. EMFCO has designed and manufactured eight unique expansion presses, controlled by state-of-the-art technology and programmable microprocessors, which are capable of machining large parts with diameters of Φ2,032mm and lengths of up to 2,438mm. Figure 15 shows a typical part of an engine machined by the company through axial loading expansion [16].
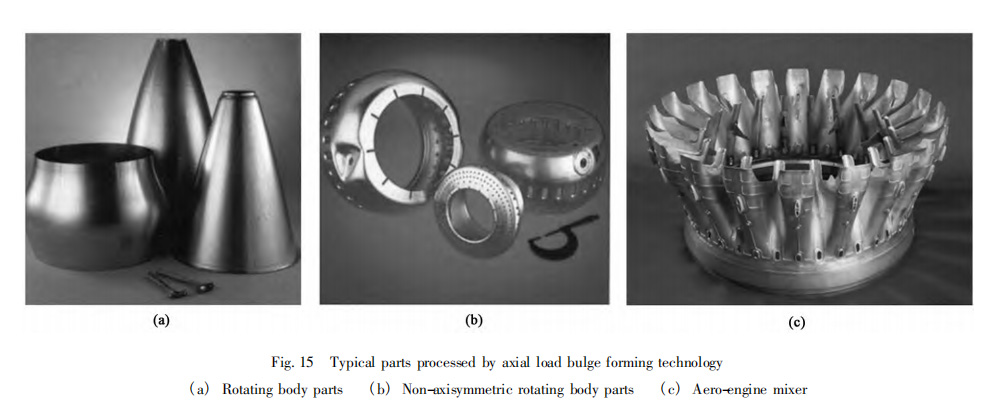
6. Multi-axial loading sheet stretch forming technology
The engine lipskin component is one of the components of an aircraft engine fairing, which covers the outer surfaces of the fairing’s intake and exhaust ports.
The lipskin component helps to smooth the airflow over the surface of the fairing, reducing drag and improving efficiency.
Figure 16 shows a prototype of a multi-axis loaded sheet drawing developed by the Advanced Metal Forming Research Team (AMFOR team) at the University of Ulster, UK, which realizes a single forming of a large-depth nacelle lip skin, which significantly reduces the fuel consumption of the aircraft after loading, as shown in Figure 17 [17].
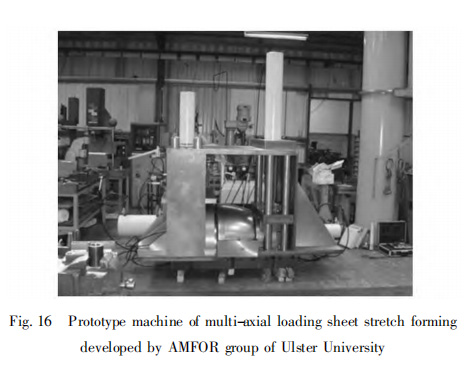
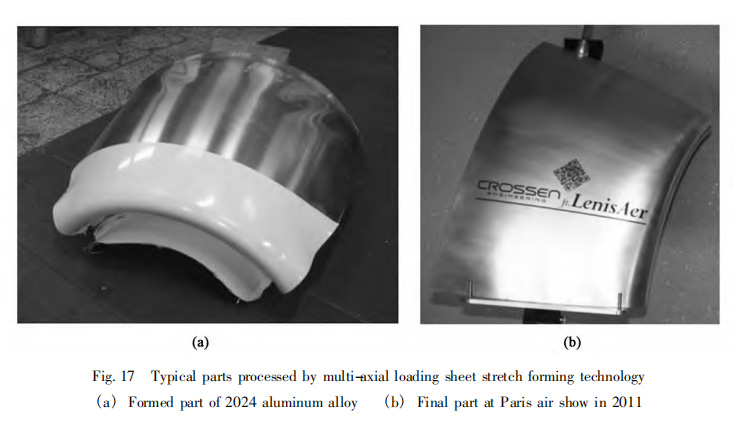
7. Hot press molding technology
The hot stamping process is divided into a non-isothermal hot stamping process and isothermal hot stamping process. Non-isothermal hot stamping process for only heating the mold or only heating the sheet forming process, isothermal hot stamping for the mold and sheet are heated to the same temperature, and then forming process. The latter is very widely used in aerospace applications [18].
Aluminum alloys of the 5000 and 6000 series increase their material elongation from 20% to 80% from 20°C to 320°C [1]. Hot press molding is a technique of plate forming that utilizes the properties of increased plasticity and elongation and decreased yield strength of the material after heating, and the forming temperature is lower than the recrystallization temperature of the material, which reduces energy consumption.
Hot press molding technology can improve the formability of the plate and obtain a uniform thickness of the part, however, it is difficult to achieve a uniform temperature of the mold during the heating process and the production cycle is longer than that of cold forming, so it is only suitable for the production of small quantities of parts. Hot press molding has the following three processes:
(1) Isothermal thermoforming, which requires preheating the mold and plate to reach the required temperature for plate forming, and then entering the press for forming. The United States Automotive Research Council will be the traditional stamping die modification for hot press molding, this process method in the mold and plate temperature control is difficult, and the mold size is not stable, forming cycle time is long.
(2) Pre-forming – heat treatment – finishing molding. The plate is preformed at room temperature, and then the preformed parts are locally heated by an induction coil for annealing treatment to remove stress, and finally formed at room temperature.
(3) Non-isothermal thermoforming. Only need to warm the plate (200 ~ 300 ℃ aluminum alloy) at room temperature mold can be formed under the full-size parts, do not need to pre-heat the mold.
France ACB, the United States CYRILBATH, and ACCUDYNE, the United Kingdom RHODES, etc. have manufactured a special hot stamping and forming equipment. Hot stamping is widely used in the forming of titanium alloy bending parts, the full use of the stress relaxation effect in the holding pressure stage can significantly improve the final forming accuracy of the part, as shown in Figure 18 [19]. By hot stamping with crimping, deep cavity-shaped parts can be formed, as shown in Figure 19 [20].
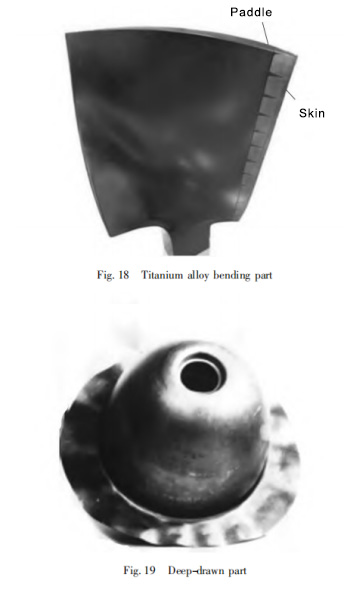
In recent years, some scholars have developed high-temperature mold change production lines [21-22].
8. Superplastic Forming / Diffusion Bonding (SPF/DB)
Some materials at a specific temperature, a certain strain rate, and under certain organizational conditions will occur superplastic phenomenon, that is, its elongation exceeds 100%. Superplastic forming is a technology that utilizes the superplastic properties of such materials to form parts, and the forming method of the sheet is superplastic pneumatic forming, i.e., compressed gas is passed into the mold or air is pumped out to form a negative pressure, so that the sheet is formed tightly against the mold [18]. It is mainly used in the aerospace field, such as the use of titanium alloy superplastic forming aircraft engine inlet ring, hatch, exhaust nozzle parts of the exhaust cone, wings, etc., aluminum alloys such as 5083 can only be used for small engine intake ring, or the use of 5083-SP part of the superplastic forming welded into the formation of an integral whole, nickel-based alloys such as Inconel 718 is being studied for the hotter parts of the engine.
Superplastic forming/diffusion connection technology is the use of materials in a certain temperature range at the same time with superplasticity and diffusion connectivity characteristics, in a cycle to complete the superplastic forming and diffusion connection, to form a complex part, increasing the strength of the parts, reducing the weight. Eurofighter’s EJ200 engine nozzle rear side of the airfoil is made of three pieces of Ti6Al4V through superplastic forming/diffusion connection molding, to improve efficiency. The rear wing of the Eurofighter’s EJ200 engine nozzle is formed from three pieces of Ti6Al4V by superplastic forming/diffusion joining, with five parts being formed at a time to increase efficiency. Rolls-Royce’s third-generation hollow-structure blades are also molded using this technique.
Boeing used friction stir welding technology to increase the size of the plate to optimize the shape of the forming blank, the use of superplastic forming method to develop the 737 airliner engine lip skin experimental parts, the material for the Ti64 titanium alloy and 5083 aluminum alloy, as shown in Figure 20 [23].
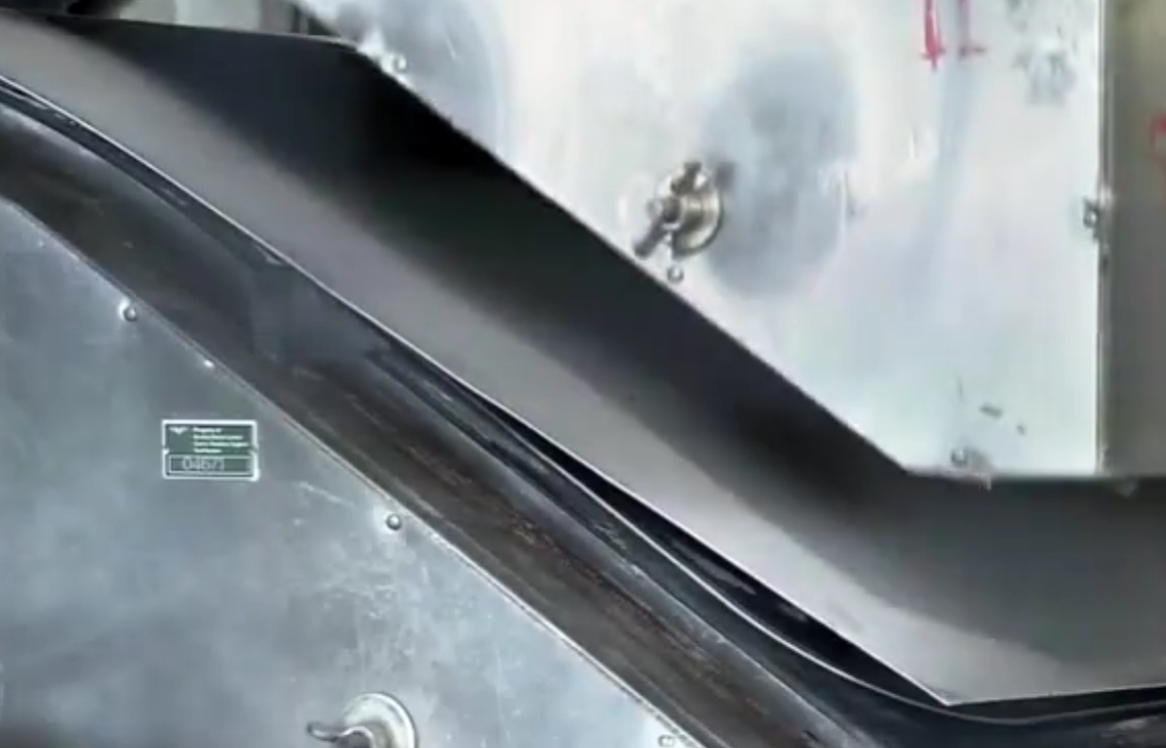
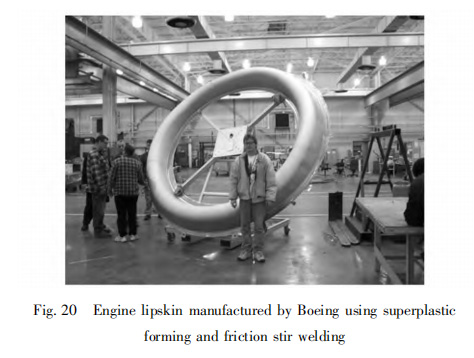
Superplastic Forming / Diffusion Bonding combination of processes for the use of materials in the superplastic state of good solid-state bonding properties and the development of a combination of process technology, it can be superplastic forming parts at the same time to complete some parts of the parts of the diffusion of the connection, to form the shape of the Very complex high-performance components. The realization of this technology has changed the traditional aircraft structural components used in riveting, screwing, gluing, and other forms, reducing the overall weight of the parts, so that the complex thin-walled parts of the whole, shorten the manufacturing cycle, improving the overall performance of the parts. Figure 21 shows the multilayer hollow structure of the engine fan blade with superplastic forming/diffusion connection, Figure 22 shows the superplastic forming equipment, Figure 23 shows the manufacturing process of the engine fan blade, and Figure 24 shows the superplastic forming/diffusion connection process of engine fan blade [24-25].
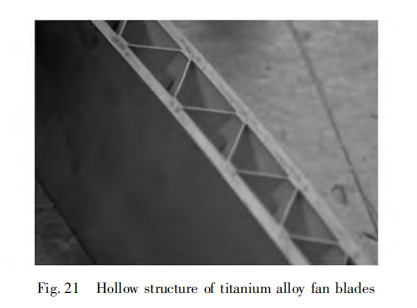
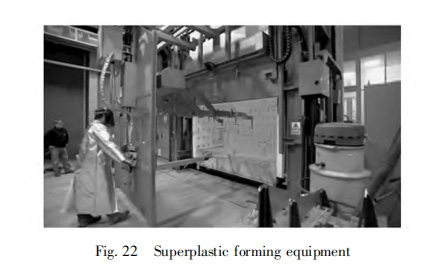
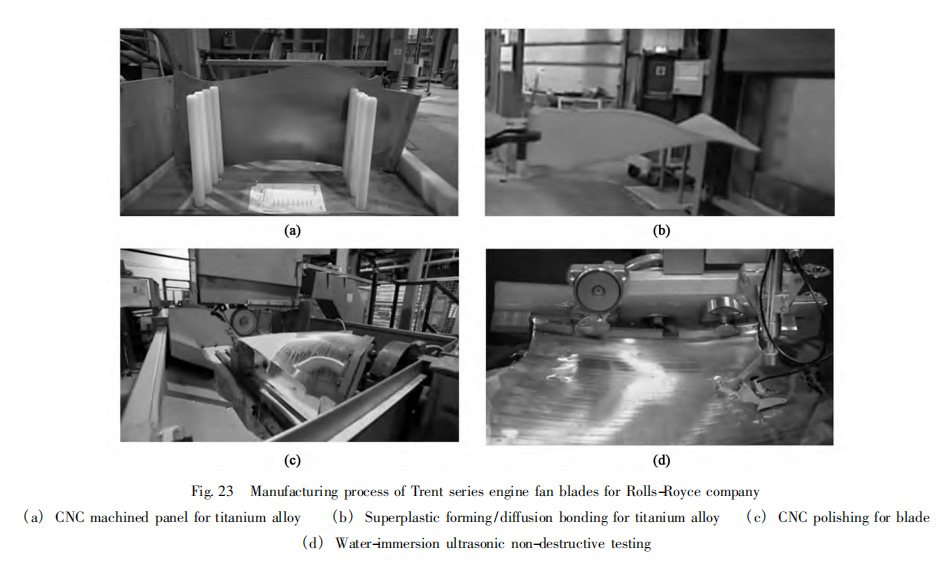
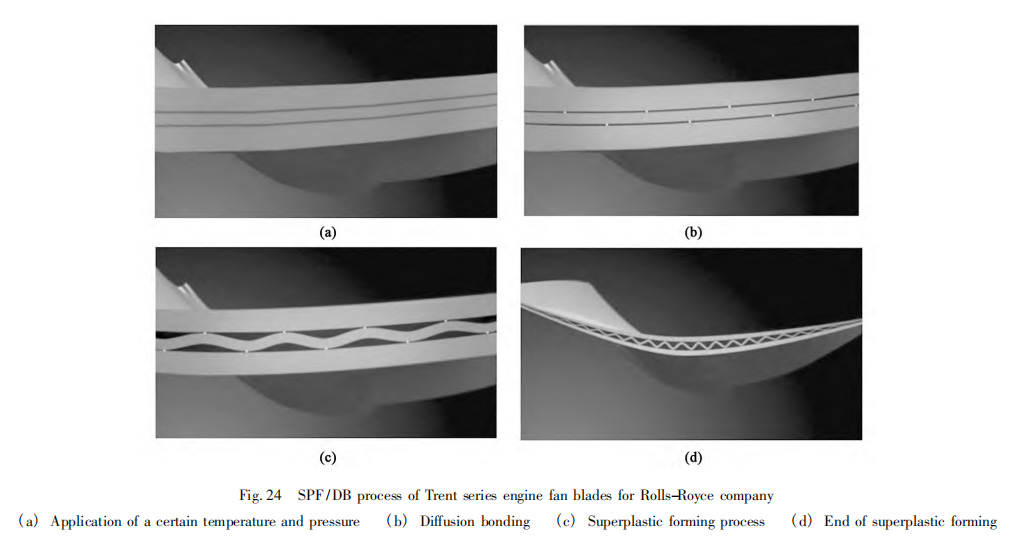
In recent years, some scholars have studied the SPF/DB technology of TC4 titanium alloy fine crystal plate, the superplastic forming and diffusion connection temperature is significantly reduced, and the superplastic forming temperature can even be reduced from 920 ℃ to 700 ℃ [26-31].
9. Hot metal gas forming technology
Hot metal gas forming technology(HMGF) is formed and developed based on hydroforming and blow molding metal high-temperature rapid prototyping technology, which makes the material form at high temperature through rapid heating, improves the forming properties of the material and improves the molding efficiency, and can realize the pneumatic forming of the complex hollow structural parts.
Hot metal gas forming uses a high-pressure gas medium to transfer the load, so that the plate or tube deforms at a certain temperature, close to the mold cavity to obtain a complex shape, with high pressure (up to 70MPa), high dimensional accuracy, wall thickness uniformity and other characteristics [32].
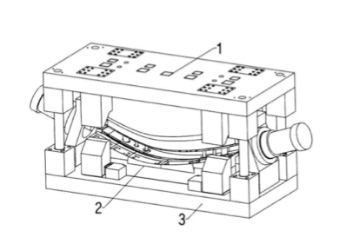
In 1999, the U.S. National Institute of Standards and Technology (NIST) and the Advanced Technology Program (ATP) carried out research funded by the “HOTMETALGASFORGING” project, the formation of the HMGF Association, in the HMGF Association to promote the HMGF process has been the rapid development of the establishment of a professional [33-34]. In 2004, European researchers started to study HMGF technology for automotive high-strength steels, typically represented by Fraunhofer IWU (Fraunhofer Institute for Tooling and Forming Technology), Thyssenkrupp AG and Linde+ Wiemann (Linde+ Wiemann). Wiemann (Linde+Wiemann) company, etc., in Asia, Japan Toyohashi University of Science and Technology, Japan Sumitomo Group, China Harbin Institute of Technology, and other successive trial production of qualified parts.
Over the past 20 years, HMGF technology after a variety of process innovations and inventions, including in the field of pressurized media, heating and sealing systems, online heat treatment and process variables, and currently in Germany, Linde Wiemann, Canada’s Multimatic (Multimatic) company, Switzerland’s Interlaken (Interlaken) company, Japan’s Sumitomo Group, etc., to achieve the industrialization of the application.
10. Free bending forming technology
Free bending forming technology was first proposed by Japanese researchers [35-36], is a new type of flexible forming technology, through the three-dimensional free bending of metal pipe, can achieve continuous changes in the bending radius of the pipe, and belongs to the moldless forming technology, the forming of complex bending components has its unique advantages. This technology can be widely used in the manufacture of aero-engine piping.
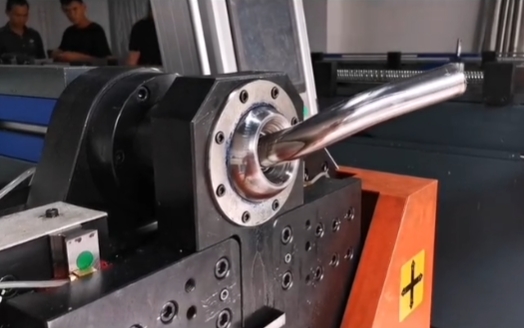
According to the different axes of forming equipment, the equipment can be divided into three-axis, five-axis, and six-axis free-bending equipment. The movement of the bending mold can be divided into two types of equipment configuration passive and active. Three-axis belongs to the passive type, five-axis, six-axis and free-bending structure based on parallel mechanism belongs to the active type [37].
At present, Japan, Germany, the United States, and the United Kingdom the researching this technology in the world’s leading position, and have been using their mastery of the three-dimensional free-bending forming principle, systematic research and development of the corresponding key technologies or three-axis, four-axis, five-axis, and six-axis free bending forming system. Although Japan has made a pioneering contribution to the field of free-form bending, the technology has not been an important engineering application in Japan. In Germany, the technology has been significantly developed.
At present, Germany has three companies were carried out by the complex components of the three-dimensional free bending forming technology and equipment for the system development and promotion of application, respectively, for the German J.NEU (Germany, Novelty) company, Thyssenkrupp (ThyssenKrupp) AG, FraunhoferIWU (Germany, the Fraunhofer Institute of molds and molding technology institute). In the U.S., Muiic has developed relevant technology and equipment, but the disclosure of the relevant technology is rarely seen, and the degree of engineering is difficult to match with the German companies, especially the German company J.NEU (Deutsche Neu-Industrie) [38].
References:
- [1] DesaiBV, DesaiKP, RavalHK. die-lessrapidprototypingprocess:Parametricinvestigations [J].ProcediaMaterialsScience,2014,6:666 -673.
- [2] Li Gaofeng. Research on the key technology of CNC double-sided progressive molding equipment design for plate materials [D]. Beijing:Beijing University of Aeronautics and Astronautics, 2019.LiGF.ResearchonKeyTechnologiesofCNCDouble-sidedIncrementalFormingEquipmentDesignforSheetMetal [D]. BeihangUniversity, 2019.
- [3] KristoffyI. Metalformingwithvibratedtools[J]. JournalofEngineeringforIndustry,1969,91(4):1168-1174.
- [4] KogaN, AsakaM, JunlapenK. Deep-drawingandironingof1050aluminumsheetsloadedwithvibrationusingNCservopressmachine[J].JournalofJapanInstituteofLightMetals,2007,57(6):240-244.
- [5] Komatsu I, Murakami T. PracticalUseofServoPress[M]. Tokyo:NikkanKougyouShinbunsha, 2009.
- [6] KimSW, LeeYS. Investigationsontheeffectofultrasonicvibrationincylindricalcupdrawingprocesses [J].KeyEngineeringMaterial,2014,622 -623:1152-1157.
- [7] Gao Tiejun, Liu Xiaojun, Yu Kun, et al. Influence of ultrasonic vibration on the tensile properties of TC1 titanium alloy sheet [J]. Rare Metal Materials and Engineering, 2019, 48(1):286-292. gaoTJ, LiuXJ, YuK, etal. EffectsofultrasonicvibrationontensilepropertiesofTC1titaniumalloysheet [J].RareMetalMaterialsandEngineering,2019,48(1):286 -292.
- [8] Han Guangchao, Liu Jun, Wen Xiaoning, et al. Ultrasound-assisted deep drawing precision molding process based on different vibration modes [J]. Forging Technology, 2021, 46(9):118-123. HanGC, LiuJ, WenXN, etal. Precisionformingprocessbyultrasonicassisteddeepdrawingbasedondifferentvibrationmodes[J]. Forging & Stamping Technology, 2021, 46(9):118 -123.
- [9]Meng De’an, Zhu Chengcheng, Dong Yuanzhe, et al. Research progress of vibration-assisted plastic forming process and mechanism [J]. Forging & Stamping Technology, 2022, 47(4):1-13. MengDA, ZhuCC, DongYZ, etal. Researchprogressonvibration-assistedplasticformingprocessandmechanism[J]. Forging & StampingTechnology,2022,47(4):1-13.
- [10] KriechenbauerS, MauermannR, MullerP. Deepdrawingwithsuperimposedlow-frequencyvibrationsonservo-screwpresses [J]. ProcediaEngineering, 2014, 81:905-913.
- [11] DunkesFirmenportraitmitPräsentationderServo-spindelpressezumTiefziehenvonBlechen [Z]. https://www.youtube.com/watch?v=d2FR. H6Jo8JQ.
- [12] Chinese Mechanical Engineering Society, Plasticity Engineering Branch, Guo Bin, Lang Lihui. Handbook of forging and pressing: Volume 2 stamping [M]. 4 edition. Beijing: Machinery Industry Press, 2021. ChinaSocietyforTechnologyofplasticity,CMES,GuoB,LangLH.HandbookofForging:Volume2Stamping[M]…4thEdition.Beijing: ChinaMachinePress, 2021. ChinaMachinePress, 2021.
- [13] ACB. ElastomerMatrixFormingPress[Z]. https://www.acbps.com/en/elastomer-matrix-forming-press-emc.
- [14] Quintus Technologies. High-pressure WarmFormingFormsAerospace-grade Titanium [Z].https://www.thefabricator.com/stampingjournal/article/stamping/high-pressure-warm-forming-formssaerospace-grade-titanium.
- [15]GB/T28273-2012,Processclassificationoftubeandsheethydroforming[S].
- [16] ExoticMetalsForming.OurProducts[Z]. https://www.exoticmetals.com/catalog/our-products.
- [17] LeacockAG.Anovelprocessforthemanufactureofextendedlaminarflowlipskins[J].KeyEngineeringMaterials,2013,549:3-12.
- [18] Li Xiaoqiang, Li Dongsheng, Du Baorui. Development of special manufacturing technology and equipment for foreign aviation sheet metal [J]. Aeronautical Manufacturing Technology, 2012,(15):32-37. LiXQ, LiDS, DuBR. Manufacturingtechnologyandequipmentsforaircraftsheetmetal[J]. AeronauticalManufacturingTechnology,2012,(15):32-37.
- [19] Shi WX, Zhang WL, Chen MH, etal. Research on multi-step hot forming process for leading edge skin of TB8 titanium alloy complex shape paddle blade[J]. Aviation Manufacturing Technology, 2021, 64(8):84-91. ShiWX, ZhangWL, ChenMH, etal.Researchonmulti- stephotformingprocessofTB8titaniumalloyleadingedgeskinofcomplexshapeblade[J]. AeronauticalManufacturingTechnology,2021,64(8):84 -91.
- [20] Liu Fei. Failure analysis and countermeasure research on deep drawing hot forming of TC1 titanium alloy thin plate [D]. Xiangtan: Xiangtan University, 2004. LiuF. heFailureAnalysesandCountermeasureStudyforDeepdrawThermalFormingofTC1TiAlloyThinPlate[D]. Xiangtan: XiangtanUniversity, 2004. XiangtanUniversity, 2004.
- [21] Shiyi Xu. Research on high temperature mold change and mold connection technology of titanium alloy hot forming [D]. Shenyang: Shenyang University of Aeronautics and Astronautics, 2020. XuSY. Research onHighTemperatureDieChangeandDieConnectionTechnologyforTitaniumAlloyHotForming[D]. Shenyang Aerospace University, 2020.
- [22] Xu Shiyi, Deng Zhonglin, Liu Chun. Design and simulation verification of high temperature mold change system for hot forming [J]. Modern Manufacturing Technology and Equipment, 2021,(2):74-76, 85. XuSY, DengZL, LiuC. Schemedesignandsimulationverificationofhightemperaturediechangingsystemforhotforming[J]. ModernManufacturingTechnologyandEquipment,2021,(2):74-76,85.
- [23]DanielSanders,PaulEdwards,GlennGrant,etal. Superplasticallyformedfrictionstirweldedtailoredaluminumandtitaniumblanksforaerospaceapplications[J]. JournalofMaterialsEngineeringandPerformance,2010,19(4):515-520.
- [24] BBCDocumentaryRollsRoyceHowtoBuildaJumboJetEngine [Z]. https://www.youtube.com/watch?v=Ho7j8OLV1fk.
- [25]Rolls-Royce. MakingaFanBlade[Z]. https://www.aerocontact.com/en/videos/12914-rolls-royce-making-a-fan-blade.
- [26] HeftiLD.Fine-graintitanium6AI-4Vforsuperplasticforminganddiffusionbondingofaerospaceproducts[J]. JOM, 2010, 62:42-45.
- [27] LuK. Stabilizingnanostructuresinmetalsusinggrainandtwinboundaryarchitectures[J].NatureReviewsMaterials,2016,1:16019. [35] Bing XI, Jianhui JU. Ying Shu, et al. Study on the influencing factors of titanium alloy superplasticity [J]. HotWorkingTechnology, 2013, 42(10):100-102. XiB, JuJH, ShuY, etal. Influencefactoronsuperplasticoftitaniumalloy [J]. HotWorkingTechnology, 2013 J]. HotWorkingTechnology,2013,42(10):100-102.
- [28] XI Bing, JU Jianhui, SHU Ying, et al. Study on factors affecting superplasticity of titanium alloy[J]. Thermal Processing Technology, 2013, 42 ( 10): 100-102. Xi B, Ju J H, Shu Y, et al. Influence factor on superplastic of titanium alloy[J]. Hot Working Technology, 2013, 42 ( 10) : 100 -J].
- [29]Zeng Liying, Zhao Yongqing, Li Danke, et al. Research progress of superplasticized titanium alloy [J]. Heat Treatment of Metals, 2005,(5):28-33. ZengLY, ZhaoYQ, LiDK, etal. esearchprogressonsuperplastictitaniumalloys[J]. HeatTreatmentofMetals. 2005,(5):28-33.
- [30] PerevezentsevVN, Chuvil’deevVN, LarinSA. Deformationmicromechanismsandsuperplasticflowrheologyinawidestrainraterange[J]. MaterialsScienceForum, 1994, 170-172(5):613-620.
- [31] SalishchevGA, GaleyevRM, ValiakhmetovOR, etal. DevelopmentofTi-6Al-4Vsheetwithlowtemperaturesuperplasticproperties[J]. JournalofMaterialsProcessingTechnology,2001,116(2-3):265-268.
- [32]Mr. Liu Gang, Mr. Wang Kehuan, Mr. He Zhubin, et al. Progress of high-pressure gas expansion molding technology for titanium alloy sheet metal components [J]. Dual-use technology and products, 2013,(6):25-28. iuG, WangKH, HeZB, etal. esearchprogressofhighpressuregasbulgingformingtechnologyfortitaniumalloysheetmetalcomponents[J]. DualUseTechnologies&Products,2013. (6):25-28.
- [33]DykstraB,PfaffmannGD,WuX. Hotmetalgasforming-Thenextgenerationprocessformanufacturingvehiclestructurecomponents[A]. ProceedingsofInternationalBodyEngineeringConference&Exposition[C]. SAE, 1999.
- [34]DykstraB. Hotmetalgasformingformanufacturingvehiclestructuralcomponents[J]. MetalForming,2001,35:50-52.
- [35] MurataM, KubokiK. CNCtubeformingmethodformanufacturing flexiblyand3-Dimensionallybenttubes[A].60ExcellentInventionsinMetalForming[C].NewYork:SpringerVerlagBerlin Heidelberg, 2015.
- [36] MurataM, MochizukiT. EffectofapproachlengthonsquaretubebyMOSbending [A].ProceedingsofAluminumAlloys. TheirPhysicalandMechanicalProperties[C]. Toyohashi:TheJapanInstituteofLightMetals,1997.
- [37]Jie Tao, Hao Xiong, Baifang Wan, et al. Three-dimensional free bending molding equipment and its key technology [J]. Precision Forming Engineering, 2018, 10(4):1-13. TaoJ, XiongH, WanBF, etal. 3Dfree-bendingformingequipmentandkeytechnology [J]. JournalofNetshapeForming Engineering, 2018, 10(4):1-13.
- [38] Ma Yannan. Research on three-dimensional free bending forming of metal pipes [D]. Nanjing:Nanjing University of Aeronautics and Astronautics, 2018.MaYN.ResearchonThree-dimensionalFreeBendingofMetalTube [D].anjing:NanjingUniversityofAeronauticsandAstronautics. 2018.
Advanced Sheet Metal Fabrication Technology by CapableMaching is licensed under CC BY-NC 4.0