Catalyzing Excellence: The Power of Plasma Electrolytic Oxidation
What is Plasma Electrolytic Oxidation
Plasma electrolytic oxidation (PEO), also referred to as electrolytic plasma oxidation (EPO) or micro-arc oxidation (MAO), is an electrochemical technique used to create oxide coatings on metal surfaces. It shares similarities with anodizing, but it involves applying higher potentials, resulting in discharges and the formation of plasma that alter the structure of the oxide layer. This process enables the growth of thick (tens or hundreds of micrometers), mostly crystalline, oxide coatings on various metals like aluminum, magnesium, and titanium. These coatings possess high hardness and provide continuous protection against wear, corrosion, heat, and electrical insulation. In plain English, it creates a ceramic coating on the surface of the metal substrate.
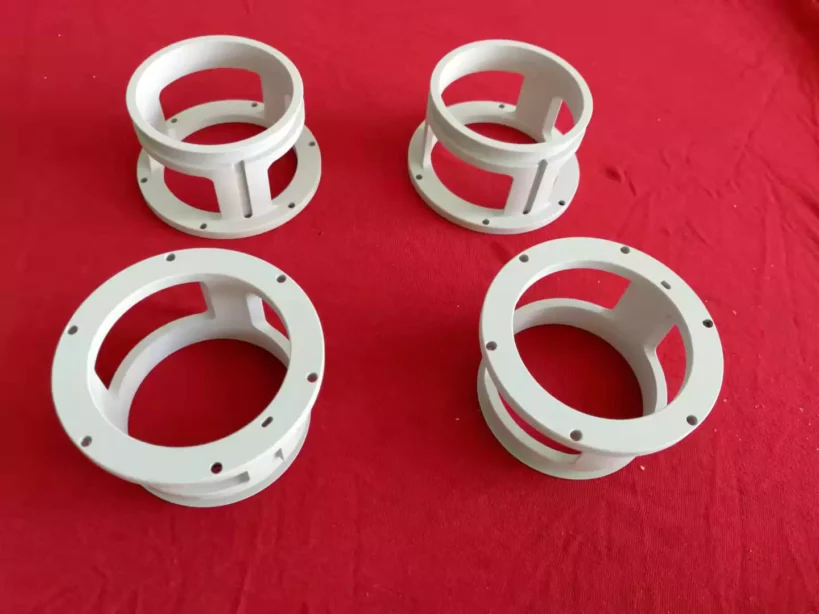
The coating formed through plasma electrolytic oxidation is a chemical conversion process that transforms the metal substrate into its corresponding oxide. This conversion occurs both inward and outward from the original metal surface. The inward growth of the coating results in strong adhesion to the substrate metal. A diverse range of substrate alloys can be effectively coated using this method, including all wrought aluminum alloys and most cast alloys. However, it’s worth noting that high silicon content in the substrate can diminish the quality of the resulting coating.
How Does the Plasma Electrolytic Oxidation Process Work
The plasma electrolytic oxidation (PEO) process involves several steps:
Preparation
The metal substrate, such as aluminum, magnesium, or titanium, is thoroughly cleaned to remove any contaminants or surface impurities. This ensures optimal coating adhesion and quality.
Electrolyte Bath
The prepared substrate is immersed in an electrolyte bath, which is typically an alkaline solution containing specific additives. The electrolyte acts as a medium for the formation of the oxide coating.
The metal substrate is connected as the anode, while a cathode, usually made of a conductive material like stainless steel, is also placed in the electrolyte bath. These electrodes are connected to a power supply.
Electric Potential Application
A controlled electric potential is applied between the anode (substrate) and the cathode. The power supply delivers a high voltage, typically in the range of hundreds of volts to several thousand volts, creating an electric field.
Microdischarge Formation
As the high voltage is applied, microdischarges or electrical sparks occur on the surface of the metal substrate. These microdischarges cause localized heating, leading to the formation of plasma.
The plasma generated during the microdischarges is highly energetic and contains ions, electrons, and reactive species. This plasma interacts with the electrolyte and the metal surface, resulting in various electrochemical reactions.
Oxide Coating Formation
The plasma electrolytic oxidation process triggers a chemical conversion of the metal substrate, where the metal combines with oxygen from the electrolyte to form the oxide coating. The coating grows both inward into the metal substrate and outward from the original surface simultaneously.
The intense energy and heat generated by the plasma during the process modify the structure of the oxide coating, making it predominantly crystalline. This results in enhanced mechanical properties, such as hardness and wear resistance. The mechanism of the formation of ceramic coatings at the microscopic level is still not fully understood. But we know the microdischarges ‘burn’ the metal surface and create ceramic coatings.
Final Rinse and Drying
Once the desired thickness and quality of the oxide coating are achieved, the substrate is removed from the electrolyte bath and rinsed to remove any residual electrolyte. It is then dried to complete the process.
Characteristics and Properties of PEO Coatings
Plasma electrolytic oxidation (PEO) coatings possess several characteristics and properties that make them advantageous for various applications. Here are some notable features:
Thickness
PEO coatings can be grown to significant thicknesses, ranging from tens to hundreds of micrometers. This allows for the formation of durable and protective layers on the metal substrate. When thickness grows, the oxide surfaces’ conductivity drops and it will take a longer time to form a further oxide layer. For instance, the first few micrometers of coating make take only minutes, it may take hours to grow a few dozen of micrometers coating.
The inward growth of PEO coatings results in excellent adhesion to the substrate metal. This strong bond enhances the coating’s stability and resistance to delamination or peeling.
Hardness
PEO coatings exhibit high hardness, typically surpassing the hardness of the base metal. This hardness contributes to improved wear resistance and durability, making them suitable for applications that require protection against abrasive forces.
Corrosion Resistance
The oxide composition of PEO coatings provides enhanced corrosion resistance to the metal substrate. The thick, continuous nature of the coating acts as a barrier, preventing corrosive substances from reaching the underlying metal and offering long-term protection against corrosion.
Thermal Resistance
PEO coatings can withstand high temperatures without significant degradation. They provide a thermal barrier that helps in dissipating heat and protecting the substrate from thermal damage or distortion.
Electrical Insulation
Due to their oxide composition and thickness, PEO coatings possess excellent electrical insulation properties. They can effectively isolate the coated metal substrate from electrical currents, making them useful for applications that require electrical insulation.
Surface Porosity
PEO coatings often exhibit a porous structure, with interconnected pores throughout the coating. These pores can be beneficial for certain applications, such as lubricant retention or enhanced bonding with other materials.
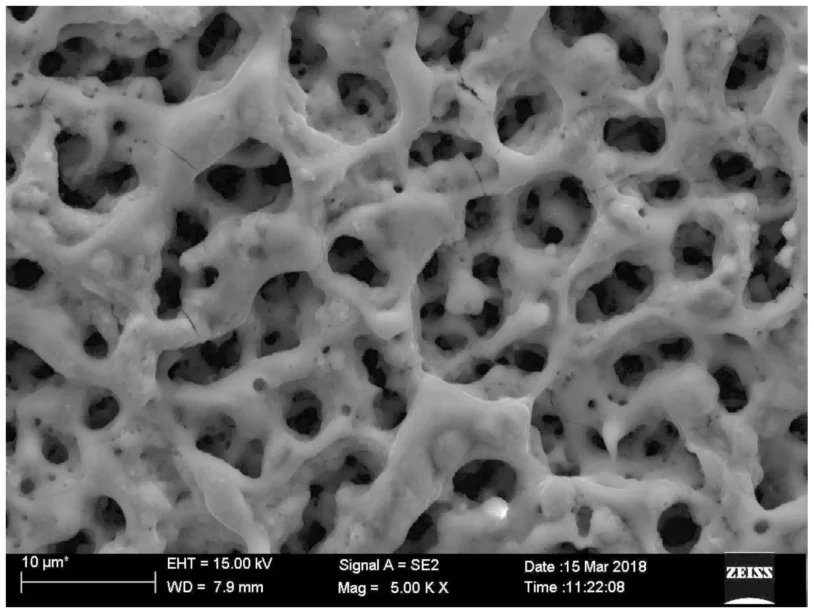
Surface Roughness
Depending on the specific PEO process parameters, coatings can exhibit varying degrees of surface roughness. This feature can be advantageous for applications where improved surface adhesion or frictional properties are required.
Aesthetics
PEO coatings can offer decorative finishes with a range of colors and appearances. By controlling the process conditions and additives, it is possible to achieve coatings with different aesthetic qualities, making them suitable for decorative or cosmetic purposes.
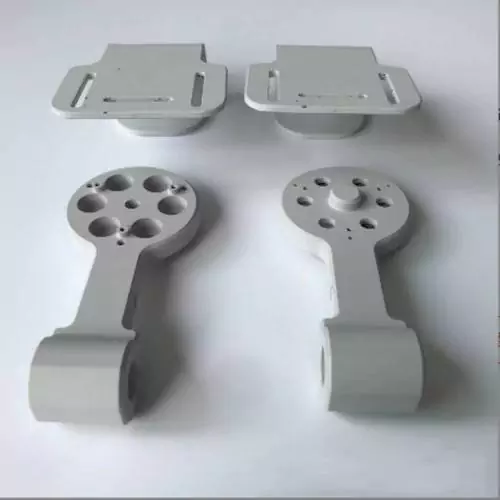
Factors Influencing PEO Process and Coating Properties
Several factors can influence the plasma electrolytic oxidation (PEO) process and the properties of the resulting coatings. These factors include:
Substrate Material
The type of metal substrate used can significantly impact the PEO process and coating properties. Different metals, such as aluminum, magnesium, or titanium, have varying reactivity, conductivity, and response to the electrolyte composition. These differences can affect the coating’s growth rate, morphology, and overall performance.
Electrolyte Composition
The composition of the electrolyte bath plays a crucial role in the PEO process and coating properties. The electrolyte contains specific additives, such as alkaline or acidic compounds, as well as salts or complexing agents. These additives can influence the growth kinetics, oxide formation, coating structure, and composition. Adjusting the electrolyte composition allows for control over coating characteristics.
Applied Voltage and Current Density
The applied voltage and current density during the PEO process have a direct impact on the coating formation. Higher voltages and current densities generally lead to faster growth rates and thicker coatings. However, excessively high voltages or current densities can cause localized overheating, sparking, or even damage to the coating.
Process Duration
The duration of the PEO process affects the coating thickness and structure. Longer processing times typically result in thicker coatings, as more oxide is formed. However, there is a limit to the duration, as prolonged processing may lead to increased porosity or other undesired effects.
Process Temperature
The temperature during the PEO process can influence the coating’s formation and properties. Higher temperatures may enhance the oxide growth rate, improve the coating’s crystallinity, and modify its composition. However, temperature control is crucial to avoid excessive heat that could lead to coating delamination or substrate damage.
Pulse Frequency and Duty Cycle
Pulsed power techniques can be employed in PEO to control the energy input and discharge behavior. The pulse frequency and duty cycle (the ratio of pulse-on time to the total pulse period) can influence the discharge intensity, energy deposition, and resulting coating properties. Optimizing these parameters can lead to desired coating characteristics.
Post-Treatment
Post-treatment processes, such as sealing, surface modifications, or heat treatments, can further enhance the properties of PEO coatings. These treatments can improve coating adhesion, increase hardness, reduce porosity, or modify the surface chemistry to achieve specific functionalities.
It’s important to note that the interplay between these factors is complex, and optimizing them requires careful consideration of the desired coating properties and the specific requirements of the application. Fine-tuning these parameters allows for tailoring PEO coatings to meet specific performance objectives.
Advantages and Limitations of Plasma Electrolytic Oxidation
Plasma Electrolytic Oxidation (PEO), or Microarc Oxidation (MAO), is a surface treatment process that produces ceramic oxide coatings on metal substrates. It offers several advantages but also has certain limitations. Let’s explore both aspects:
Advantages of PEO:
Enhanced Surface Properties
PEO can significantly improve the surface properties of metal substrates. The process forms a dense, hard, and wear-resistant ceramic oxide layer on the surface, which can provide increased hardness, corrosion resistance, and thermal stability compared to the base material.
Improved Wear Resistance
PEO coatings exhibit excellent wear resistance due to their high hardness and low friction characteristics. This makes them suitable for applications in industries such as automotive, aerospace, and machinery, where components experience high levels of wear and friction.
Corrosion Protection
PEO coatings can effectively protect metal substrates from corrosion. The ceramic oxide layer acts as a barrier, preventing the underlying metal from coming into contact with corrosive substances. This makes PEO coatings suitable for applications in aggressive environments.
Thermal Barrier Properties
PEO coatings can also provide thermal insulation to the underlying metal substrate. This is especially useful in applications where there are significant temperature gradients, as the coating can help reduce heat transfer and improve thermal stability.
Limitations of PEO:
Complexity and Cost
PEO is a complex and specialized process that requires advanced equipment and expertise. The equipment setup and maintenance can be costly, making it less accessible for small-scale operations. The process can also be time-consuming, adding to the overall production costs.
Size and Geometry Limitations
PEO is typically limited to small to medium-sized components due to the size restrictions of the equipment. The process may not be suitable for larger or complex-shaped parts that cannot fit into the PEO setup.
Process Variability
Various parameters, such as electrolyte composition, current density, and process duration can influence PEO. Achieving consistent and repeatable results across different batches or materials can be challenging, requiring careful process control and optimization.
Substrate Limitations
PEO is primarily applicable to metallic substrates, and the effectiveness of the process depends on the specific properties of the base material. Not all metals or alloys may be suitable for PEO, limiting their applicability to certain materials.
It’s important to note that the advantages and limitations of PEO can vary depending on the specific application, process parameters, and desired outcomes. Proper evaluation and understanding of these factors are crucial for determining the suitability and feasibility of PEO for a given situation.
Typical Application
Small piston engines are probably the most typical use of MAO. In order to have a very lightweight, Instead of using steel, some small piston engines apply aluminum with MAO. It resists heat, extremely abrasion-resistant. Of course, there are much more applications of MAO in various industries.
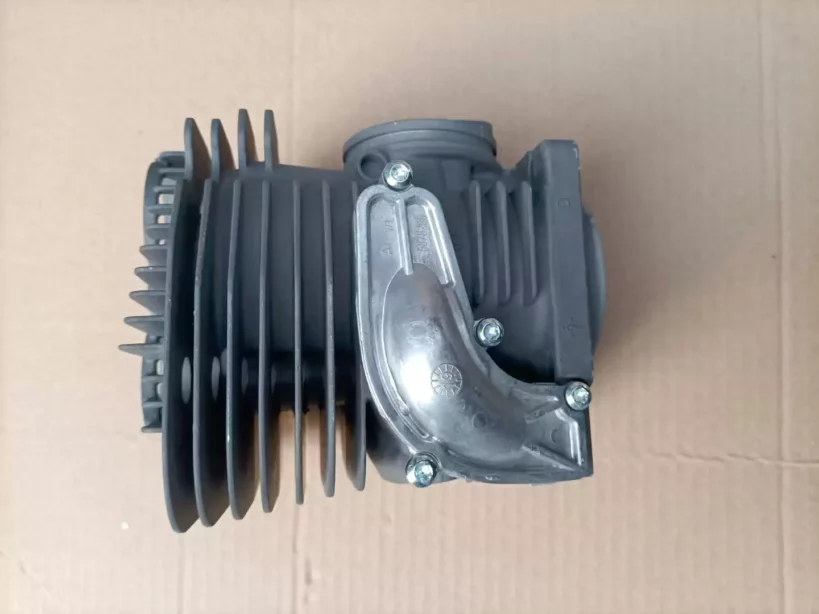
Summary
The PEO process offers several advantages. It enhances the surface properties of metal substrates, providing increased hardness, wear resistance, corrosion protection, and thermal stability. The resulting coating can withstand harsh environments and reduce friction. PEO coatings find applications in industries such as automotive, aerospace, and machinery, where improved surface properties are crucial.
The PEO process, however, has some limitations. It can be complex and expensive, requiring specialized equipment and expertise. Size and geometry limitations exist, limiting its applicability to smaller and medium-sized components. Achieving consistent results across different batches or materials can be challenging, necessitating careful process control and optimization.
In summary, PEO is a valuable surface treatment process that can enhance the properties of metal substrates. It provides improved hardness, wear resistance, corrosion protection, and thermal stability. While it has some limitations, PEO is widely used in various industries where superior surface properties are desired.