Exploring the Power of Powder Metallurgy
Powder metallurgy (PM) encompasses various methods for creating materials or components using metal powders. By employing PM processes, the reliance on subtractive manufacturing techniques can be minimized or eliminated altogether. This not only decreases material waste but also leads to cost savings in the production of the end product.
What is powder metallurgy?
Powder metallurgy serves as a means to produce unique materials that are difficult or impossible to obtain through melting or other conventional methods. One notable example is tungsten carbide (WC), a crucial product in the industry. WC, composed of bonded WC particles and cobalt, is extensively utilized for cutting and shaping various metals.
Additionally, powder metallurgy yields a diverse range of products, including sintered filters, porous oil-impregnated bearings, electrical contacts, and gears. These products find widespread use across different industries.
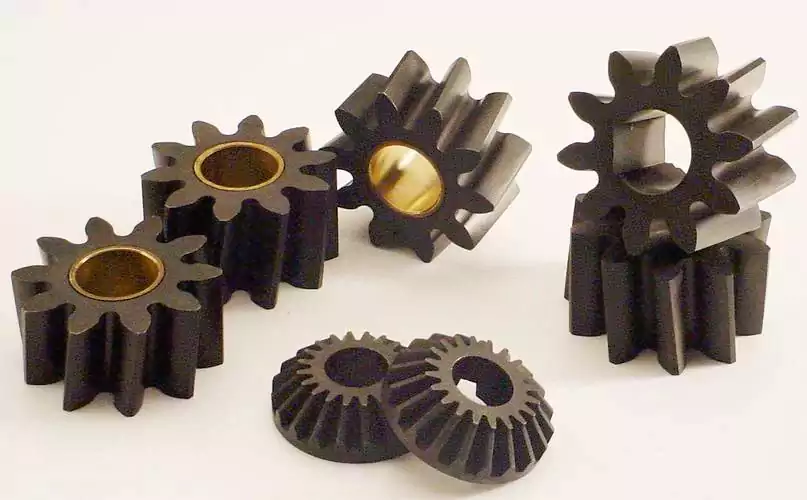
In recent years, the introduction of large-scale metal powder-based additive manufacturing (AM) techniques, such as selective laser sintering, has emerged as a new and commercially significant category within powder metallurgy applications. This development has opened up further possibilities for the production of complex components and objects using metal powders.
Powder Metallurgy Process
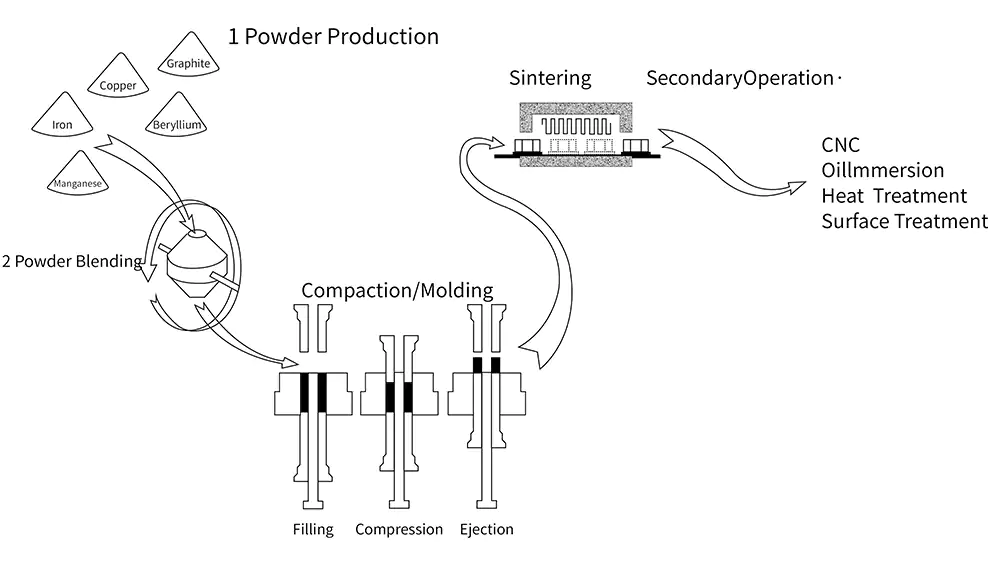
The powder metallurgy process involves several key steps to transform metal powders into finished components. Here is an overview of the typical powder metallurgy process:
Powder Production
Metal powders are produced through methods such as atomization, mechanical alloying, or chemical processes. This stage ensures the liquid metal powders have the desired composition and properties.
Powder Blending
Different powders may be mixed to achieve specific alloy compositions or to enhance certain properties. Powder blending ensures a homogeneous mixture before further processing.
Compaction
The blended powders are placed into a die cavity and subjected to high-pressure compaction. This process applies force to compact the powders, creating a green compact with the desired shape and density.
Sintering
The green compact is heated in a controlled atmosphere or vacuum to a temperature below the melting point of the metal. Sintering causes the particles to bond, resulting in densification and the formation of a solid component with improved mechanical strength.
Secondary Operations
Additional treatments may be applied to enhance the properties or surface finish of the sintered part. These operations can include machining, heat treatment, impregnation, coating, or surface modification.
Inspection and Quality Control
The finished components undergo a rigorous inspection to ensure they meet the required specifications. This may involve dimensional measurements, microstructural analysis, mechanical testing, and non-destructive testing.
Finishing
If necessary, the components may undergo further finishing operations such as polishing, plating, or coating to achieve the desired aesthetic or functional properties.
The powder metallurgy process offers advantages such as cost-effectiveness, material efficiency, complex geometries, and the ability to produce parts with tailored properties. It finds applications in various industries, including automotive, aerospace, electronics, medical, and consumer goods.
Powder Metallurgy Methods
Powder metallurgy encompasses various methods for manufacturing components from metal powders. Here are some common powder metallurgy methods:
Pressed Powder Metallurgy
Pressed powder metallurgy, also known as powder compaction or powder pressing, is a manufacturing method that involves the compaction of metal powders into desired shapes using high-pressure equipment. The process begins with the selection and blending of metal powders, followed by the placement of the powders into a die cavity. A high-pressure force is applied to compact the powders, resulting in a green compact with the desired shape and density. The green compact is then subjected to sintering, where the powders bond together to form a solid component.
Metal Injection Molding
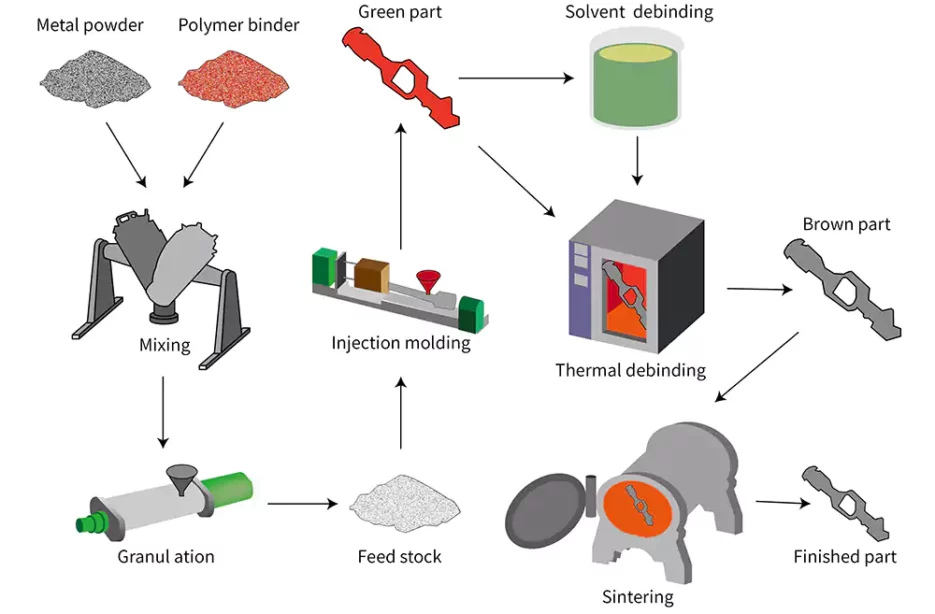
Metal injection molding (MIM) is invented by Parmatech in USA in 1973. In the early 1980s, many companies in Europe and Japan made significant efforts to research and rapidly popularize this technology. Particularly in the mid-1980s, the technology made significant advancements after industrialization and experienced astonishing annual growth rates.
Globally, there are now over 100 companies involved in the development and sales of this technology. MIM technology has become a vibrant high-tech field in the new manufacturing industry. It is a pioneering technology in the global metallurgical industry and a major direction for the development of powder metallurgy technology.
MIM technology, which combines various disciplines such as plastic molding, polymer chemistry, powder metallurgy, and materials science, enables the rapid and precise manufacturing of high-density, high-precision, three-dimensional composite-shaped components using mold injection molding. It allows the transformation of design ideas into products with specific structures and functional characteristics and facilitates the mass production of components. It represents a new paradigm in the manufacturing technology industry.
Metal injection molding (MIM) is an advanced metalworking process that involves combining finely-powdered metal with a binder material to create a specialized “feedstock.” The most common binder material is a thermoplastic polymer such as PP, PE, PS, or sometimes Wax. This feedstock is then shaped and solidified using injection molding techniques. The process allows for the efficient production of high-volume and complex parts in a single step.
Theoretically, MIM can be utilized for large parts, but in industries, MIM technology is mostly utilized for large quantity small parts. MIM feedstock can consist of various metals, with stainless steel being the most common choice due to its widespread use in powder metallurgy.
After the initial molding stage, the binder material in the feedstock is removed through a process called de-binding. The most common de-binding is done by heating the molded parts so that the binder can be extracted through the small channels in the molded parts. Subsequently, the metal particles undergo diffusion bonding and densification to achieve the desired strength properties. Notably, this densification process typically leads to a shrinkage of approximately 15% ~25% in each dimension of the final product.
Once the debinding process is complete, the remaining binder-free part called the brown part, is subjected to a high-temperature sintering process. Sintering involves heating the part in a controlled atmosphere, causing the metal particles to bond together and densify. The part undergoes shrinkage during this process, resulting in further compaction.
After sintering, the part may undergo additional finishing operations, such as machining, polishing, or surface treatment, to achieve the desired final shape, dimensions, and surface characteristics. The typical density achieved after the Metal Injection Molding (MIM) process is generally high and close to the theoretical density of the metal material used. In general, the density of MIM parts can range from 95% to 99.8% of the theoretical density. Achieving densities above 98% is common in many MIM applications.
MIM has found applications in numerous industries, producing small components for a wide range of products. By leveraging the benefits of MIM, manufacturers can achieve cost-effective production, intricate designs, and improved mechanical properties.
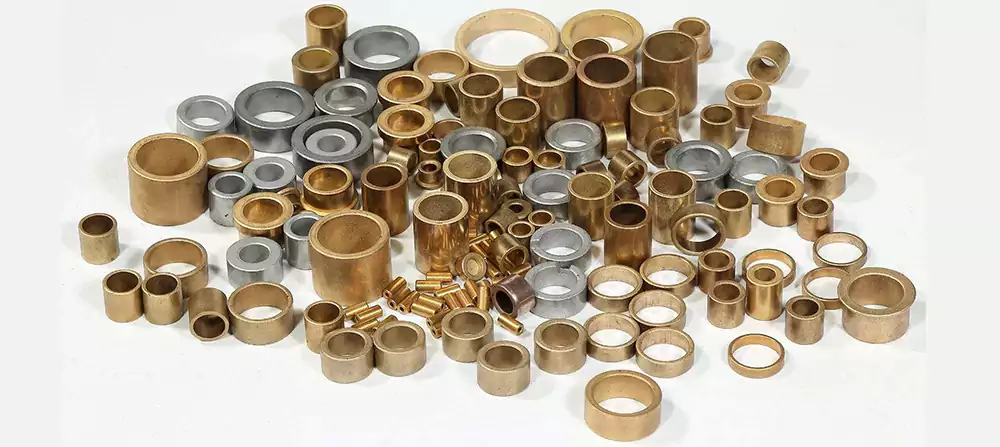
Electric Current Assisted Sintering
Electric current-assisted sintering (ECAS) technologies rely on electric currents to densify powders, with the advantage of reducing production time dramatically (from 15 minutes of the slowest ECAS to a few microseconds of the fastest), not requiring a long furnace heat and allowing near theoretical densities but with the drawback of simple shapes. Powders employed in ECAS can avoid binders thanks to the possibility of direct sintering, without the need for pre-pressing and a green compact.
Molds are designed for the final part shape since the powders densify while filling the cavity under applied pressure thus avoiding the problem of shape variations caused by nonisotropic sintering and distortions caused by gravity at high temperatures. The most common of these technologies is hot pressing, which has been under use for the production of the diamond tools employed in the construction industry. Spark plasma sintering and electro-sinter forging are two modern, industrial commercial ECAS technologies.
Advantages of Powder Metallurgy
Powder Metallurgy (PM) offers several advantages as a manufacturing process for producing metal components. Here are some of the key advantages:
Cost-effectiveness
Powder metallurgy can be a cost-effective method compared to traditional manufacturing processes like machining or casting. It allows for high material utilization since little to no material is wasted during the process. Additionally, PM can reduce the need for extensive machining operations, which further reduces costs.
Complex geometry
PM enables the production of components with complex shapes and intricate features that may be challenging or costly to manufacture using other methods. The flexibility of the powder metallurgy process allows for the creation of components with internal cavities, undercuts, and fine details.
Material versatility
Powder metallurgy is applicable to a wide range of materials, including various ferrous and non-ferrous metals, as well as ceramics and composites. This versatility allows for the production of components with diverse properties and characteristics to meet specific application requirements.
Enhanced material properties
The PM process can result in components with excellent mechanical properties. During the compaction and sintering stages, the metal powders bond together, leading to a dense and homogenous structure. This results in components with high strength, good wear resistance, and improved dimensional stability.
Control over porosity
Porosity control is one of the significant advantages of PM. By adjusting the powder characteristics, compaction parameters, and sintering conditions, it is possible to control the porosity of the final component. This allows for tailored porosity levels, which can be advantageous in applications where porosity is desired, such as for self-lubricating or filtration components.
Reduced waste and environmental impact
Powder metallurgy is a near-net-shape manufacturing process, meaning it produces components that require minimal additional machining or processing. This reduces waste and energy consumption, leading to a lower environmental impact compared to conventional manufacturing methods.
Improved material utilization
PM allows for the use of recycled materials and waste materials generated from other processes. These materials can be blended with new powders to produce components, promoting sustainability and reducing raw material costs.
Consistency and repeatability
Powder metallurgy offers excellent control over the manufacturing process, resulting in consistent and repeatable product quality. This is particularly beneficial for industries that require high precision and reliability, such as the automotive, aerospace, and medical sectors.
Applications of Powder Metallurgy
Powder metallurgy (PM) finds applications in various industries due to its versatility and ability to produce components with specific properties. Here are some common applications of the powder metallurgy technology:
Automotive Industry
Powder metallurgy is extensively used in the automotive industry for manufacturing components such as gears, camshafts, connecting rods, valve seat inserts, sprockets, and oil pump rotors. PM components offer high strength, wear resistance, and dimensional accuracy, making them suitable for demanding automotive applications.
Aerospace Industry
The aerospace industry utilizes several powder metallurgy processes for manufacturing critical components like turbine blades, compressor parts, and structural components. PM components in aerospace applications provide high-temperature resistance, corrosion resistance, and lightweight characteristics, contributing to improved aircraft performance.
Electronics Industry
Powder metallurgy is used in the electronics industry for manufacturing electrical contacts, magnetic components, heat sinks, and connectors. PM components provide good electrical conductivity, magnetic properties, and thermal management capabilities.
Power Tools and Appliances
Powder metallurgy is employed in the production of components for power tools, appliances, and household items. Examples include gears, bearings, bushings, and cutting tools. PM components in these applications offer high wear resistance, dimensional stability, and improved efficiency.
Oil and Gas Industry
Powder metallurgy is utilized in the oil and gas industry for manufacturing components like valve bodies, pump parts, and drilling tool components. PM components in this sector provide high strength, corrosion resistance, and the ability to withstand harsh operating conditions.
Defense and Firearms Industry
Powder metallurgy is used in the defense sector for producing firearm components, such as gun barrels, firing pins, and breechblocks. PM components in firearms offer improved strength, durability, and dimensional precision.
Conclusion
In conclusion, powder metallurgy (PM) is a highly versatile manufacturing process that offers numerous advantages in the production of metal components. It enables the creation of complex shapes, precise dimensional control, and the utilization of a wide range of materials.
Overall, powder metallurgy is a valuable manufacturing technique that continues to play a significant role in the production of high-quality metal components, contributing to advancements in various industries and meeting the demands of modern engineering challenges.